Introduction:-
Azolla is a branched free-floating aquatic fern, and it rapidly grows on the surface of the water. Many farmers due to Limited resources they often struggle to produce sufficient feed for those animals. But Azolla is the right option in front of them.
Azolla is an ideal sustainable feed for cattle, fish, pigs, and poultry apart from this its also used as biofertilizer on the farm. Hence many farmers attract to the Azolla cultivation.
Azolla cultivation is popular in countries like China, Vietnam, and the Philippines, etc.
Azolla fixes nitrogen it is an excellent source of nitrogen also it to his high nutrient value.
Azolla cultivation required less amount of investment hence it is a low-cost alternative for a good feed and good biofertilizer.
selection and cleaning of the dome:-select the dome from Vigyan Ashram campus then clean the inside and outside surface of the dome properly remove break and unwanted part from the dome
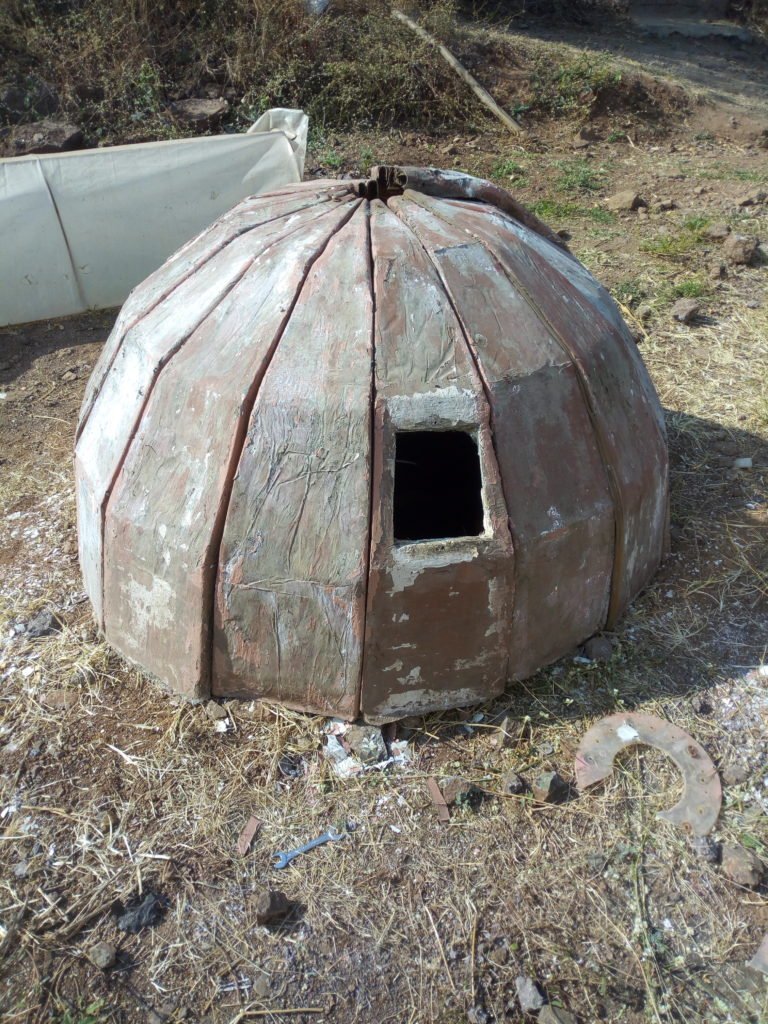
measure the dimension of dome:- base diameter of dome=146cm radius of dome=73cm height of dome=83cm total area of dome=3.3m^2 volume of dome=0.81m^3
repairing of dome:- selected dome condition is very bad and so many nut and bolt are missing. I had insert new nut bolt at missing position
design and installation top sheet of the dome:– the upper surface sheet of dome was breaked so I had creat a new upper sheet. the dimension of sheet such as outer diameter of sheet=25.1cm inner diameter of sheet=12.6 thickness of sheet=0.6cm
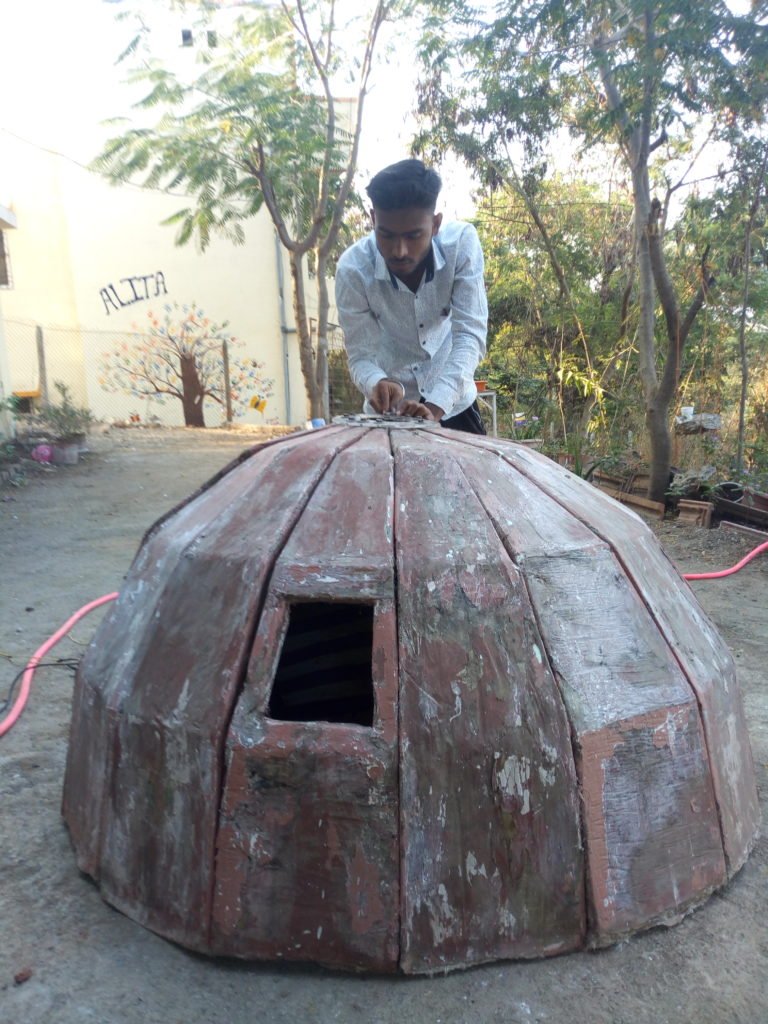
Dimensions 0f tray:- diameter of 1st tray=1.35m diameter of 2nd tray=1.15m area of 1st tray=1.4m^2 area of 2nd try=1.05m^2 total volume of 1st tray=0.084^3 total volume of 2nd tray=0.062m^3 water volume in 1st tray=1.41lit/mm water volume in 2nd tray=1.05lit/mm
calculation:-
F=PXA where F=force, P=pressure A=area. hydrostatic pressure(p)=ρgh where ρ=density of water, g=accelaration due to gravity, h=height of water level. p=2ST/D where p=pressure in kpa, D=diametre in m, T=wall thickness in m, S-allowable stress in mpa(F/A).
fabrication of F.R.P tray :
Information about F.R.P :
Fiber-reinforced plastics (FRP), also known as fiber-reinforced polymers, are a category of composite plastics specifically using fiber materials to mechanically augment the elasticity and strength of the plastic. They consist of a polymer matrix – the original plastic which is usually tough but weak – which is blended with a reinforcing material to yield a final product with the desired material or mechanical properties
Procedure of fabrication of tray :
- initially discussion with sonal mam 0n which type of material is used for tray
- then we got detail knowledge about different material properties
- becouse it is used water collection for azolla plantation thus it is always contact with water
- therefor firstlycorrosion problem is defined, the overcome this problem “F.R.P” material is selected for fabrication of tray
- then take it’s dimension on the basic of dimeter of dome
- the upper layer of circular tray dimeter is 135 cm and lower of tray dimater is 115 cm and height of side edge of tray is 6 cm
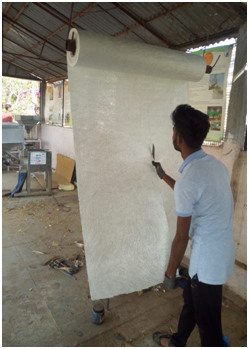
- Then draw the sketch of circular tray and it is divided into four part
- Then purches the required material
- Then cut F.R.Psheet in required dimension for making of 1st and 2nd tray and they are place over the glass table
- Then gives 200 ml resin 2 ml cobalt and 2 ml hardner into the biker and then they are properly mixed each other
- Then this mixture is apply over the F.R.Psheet
- The total quantity of applying resin 1200ml, cobalt 12ml, and hardner 12ml for making of 1st and 2nd tray
- this is required one day for drying
- After drying sheet join the side edge of tray
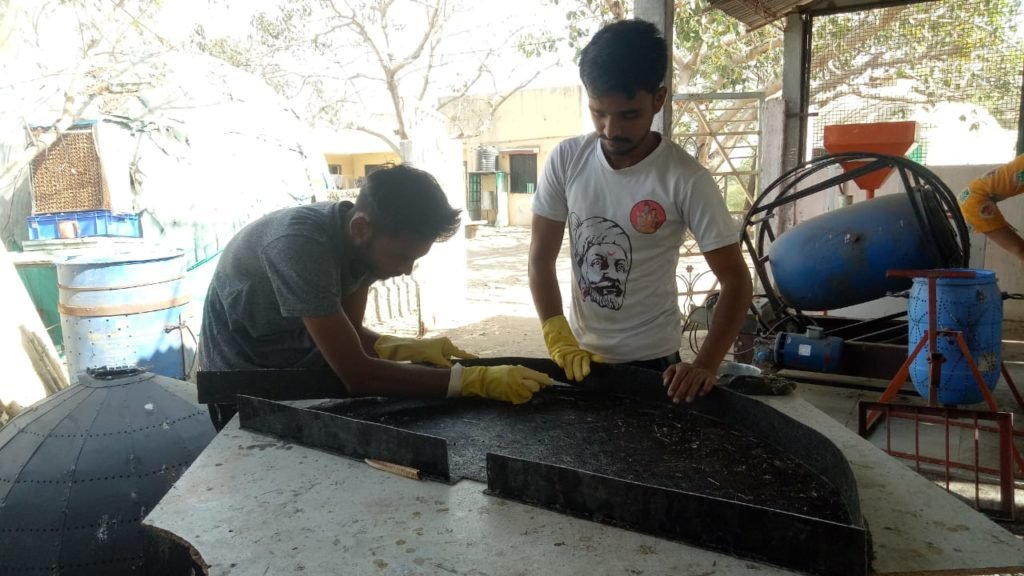
- Making 1st and 2nd tray required 2 days
- So that This similar procedure is required for making of remening tray
Installation of tray under the dome:
Initially clean and plane the ground surface where tray is mounded, then stand is installed in plane surface. The already fabricated stand is taken from sunny sir. Then tray is mounted over the stand
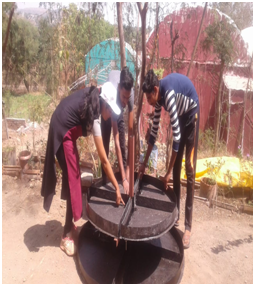
4.2.8Information about LEDs
Light-emitting diodes (LEDs) are semiconductors that convert electrical energy into light energy. The color of the emitted light depends on the semiconductor material and composition, with LEDs generally classified into three wavelengths: ultraviolet, visible, and infrared.
The wavelength range of commercially available LEDs with single-element output power of at least 5 mW is 360 to 950 nm. Each wavelength range is made from a specific semiconductor material family, regardless of the manufacturer. This article will provide an overview of the LED operation and a brief look at the industry. Various types of LEDs, the corresponding wavelengths, materials used in their composition, and some applications for the specific lamps will also be discussed.
Ultraviolet LEDs (UV LEDs): 240 to 360 nm
UV LEDs are specifically used for industrial curing applications, water disinfection, and medical/biomedical uses. Power output levels greater than 100 mW have been achieved at wavelengths as short as 280 nm. The material primarily used for UV LEDs is gallium nitride/aluminum gallium nitride (GaN/AlGaN) at wavelengths 360 nm or longer. Shorter wavelengths utilize proprietary materials. While the market for wavelengths 360 nm and longer is stabilizing because of lower prices and plentiful suppliers, shorter wavelengths are manufactured by only a few suppliers, and the prices for these LEDs are still very high compared with the rest of the LED product offerings.
Near-UV to green LEDs: 395 to 530 nm
The material for this wavelength range of products is indium gallium nitride (InGaN). While it is technically possible to make a wavelength anywhere between 395 and 530 nm, most large suppliers concentrate on creating blue LEDs (450 to 475 nm) for producing white light with phosphors, and green LEDs in the 520- to 530-nm range for traffic signal green lighting. The technology for these LEDs is generally viewed as mature. Improvements in optical efficiency have slowed down or stopped over the last few years.
Yellow-green to red LEDs: 565 to 645 nm
Aluminum indium gallium phosphide (AlInGaP) is the semiconductor material used for this wavelength range. It is predominately made in traffic signal yellow (590 nm) and traffic signal red (625 nm). The lime-green (or yellowish-green 565 nm) and orange (605 nm) are also available in this technology but have limited availability.
It is interesting to note that neither the InGaN nor AlInGaP technologies are available as a pure green (555 nm) emitter. Older, less efficient technologies do exist in this pure green region but are not considered efficient or bright. This is due largely to a lack of interest/demand from the marketplace and therefore a lack of funding to develop alternative material technologies for this wavelength region.
Deep red to near-infrared: 660 to 900 nm
There are many variations on device structure in this region, but all use a form of aluminum gallium arsenide (AlGaAs) or gallium arsenide (GaAs) materials. Applications include infrared remote controls, night-vision illumination, industrial photocontrols and various medical applications (at 660–680 nm).
Artiftcial light source for photosynthesis
Artificial lighting should provide plants with energy and information required for development. For this purpose, fluorescent lamps, particularly those having enhanced blue and red spectra (i.e. cool fluorescent white lamps), are widely used in growth chambers, together with additional light sources to achieve the sustained photosynthetic photon fluence necessary for high productivity. However, the spectrum and intensity of fluorescent lights are not stable over a long time (see the comparative information in the electronic supplementary material).
High intensity discharge (HID) lamps, such as metal halide and high-pressure sodium lamps, have relatively high fluence (max. 200 lumens per watt) and high photosynthetically active radiations (PARs) efficiency (max. 40%), and are typically used in greenhouses and plant growth rooms. The drawbacks including elevated arc to fire energy requirement, the high operational temperature preventing placement close to the canopy and the spectral distribution (high proportion of green–yellow region, significant ultraviolet radiation and altered red : far red ratio), which may shift according to the input power, strongly limit their use and innovation. Among artificial lighting systems, LEDs present the maximum PAR efficiency (80–100%; see the electronic supplementary material). LEDs emitting blue, green, yellow, orange, red and far red are available and can be combined to provide either high fluence (over full sunlight, if desired), or special light wavelength characteristics, thanks to their narrow-bandwidth light spectrum. The high efficiency, low operating temperature and small size enable LEDs to be used in pulsed lighting and be placed close to the leaves in interlighting and intracanopy irradiation. Their long life expectancy and ease of control make them ideal for greenhouses in use all year round. The LED technology is predicted to replace fluorescent and HID lamps in horticultural systems and to revolutionize controlled growth environments.
Changing light intensity and quality
From the biological point of view, the main questions about LEDs are related to their ability to mimic and enhance the beneficial effects of natural light while avoiding the adverse influence. Below, selected examples are used to provide a short review on useful properties of LED lights in these aspects.
Light-emitting diode light(s) can sustain normal plant growth
Pioneer experiments on plant growth under red LEDs on lettuce were reported by Bula et al.Martineau et al.calculated that the amounts of dry matter per mole of artificial lighting gained by lettuce grown using red (650 nm) LEDs or high-pressure sodium lamps were identical, and Chang et al. calculated that the maximum photon utilization efficiency for growth of the green alga Chlamydomonasreinhardtii under red LEDs is centred at 674 nm. Lettuce grown under red LEDs presented hypocotyls and cotyledons that were elongated, a phenomenon known to be phytochrome-dependent. Under red LEDs illumination, phytochrome stimulation is especially high as far red light is not provided. Hypocotyl elongation could be prevented by adding at least 15 µmol m–2 s−1 of blue light. Although a complete demonstration was not provided, one can hypothesize that the supplemented blue light activated cryptochrome, a blue-light photoreceptor that mediates reduction of hypocotyl length.
The efficiency of red (650–665 nm) LEDs on plant growth is easy to understand because these wavelengths perfectly fit with the absorption peak of chlorophylls and phytochrome, while the supplemented blue light introduced the idea that growth under natural light could be mimicked using blue and red LEDs. In addition to providing a better excitation of the different types of photoreceptors, the blue + red combination allowed a higher photosynthetic activity than that under either monochromatic light. Some authors attributed this effect to a higher nitrogen content of the blue-light-supplemented plants, whereas others suggested a better stomatal opening, thus providing more CO2 for photosynthesis. It is well established that stomata opening is controlled by blue-light photoreceptors. This is possibly reflected in the increase of shoot dry matter with increasing levels of blue light. The supplementation of blue + red LEDs could also be complemented with green LEDs. Illumination with more than 50% of green LED light causes a reduction in plant growth, whereas treatments containing up to 24% green light enhanced growth for some species. Recently, LEDs have been successfully tested for their ability to allow the growth of agronomically important crops, fruit and flower plants, and even trees. Table 1shows the parameter changes in selected taxa exposed to different wavelengths of LEDs compared with the other light sources.
Chloroplast differentiation and de-differentiation
In the absence of light or under deep shade conditions, plants develop etiolation symptoms, such as the absence of Chl, reduced leaf size and hypocotyl elongation. When the plants are exposed to light, chloroplast differentiation involves the accumulation of proteins, lipids and photosynthetic pigments. The kinetics of Chl accumulation present a lag phase under white LED light, which is eliminated when plants are grown under blue LED (460–475 nm) but not in red LED light (650–665 nm). Interestingly, similar Chl amounts were reached, regardless of the LED colour. In contrast to Chl, red LED-irradiated pea leaves contained higher levels of β-carotene than those grown under blue or white LED light. The light intensity is also important in Chl synthesis. For instance, Tripathy& Brown showed that wheat seedlings accumulated Chl under red LED light at 100 µmol m–2 s–1, but not at 500 µmol m–2 s–1. This inhibition of Chlaccumulation under high fluence red LED light could be avoided by the supplementation of blue light (30 µmol m–2 s–1). Although no demonstration of the effect was provided by the authors, the absence of Chl accumulation under high fluence red light could result from a fast photodestruction of the newly formed Chl molecules. Interestingly, re-etiolation provides adequate conditions for the production of white asparagus, chicory or seakale. In tea leaves, the re-etiolation increases the content of volatiles (aroma), especially volatile phenylpropanoids/benzenoids and several amino acids, including l-phenylalanine, suggesting the activation of a plastid-located shikimate pathway.
High fluence light-emitting diode triggers production of secondary compounds
Photosynthetic organisms exposed to high light develop short- and long-term response mechanisms to reduce stress effects. Some of these mechanisms are the specific topic of other papers included in this special issue (xanthophyll cycle, non-photochemical quenching, re-oxidation of the reduction equivalents through photorespiration, the malate valve and the action of antioxidants). This section is dedicated to the metabolic shifts triggered by high light stress. They are used in repairing mechanisms, shielding, reactive oxygen species (ROS) quenching or the production of storage compounds. The synthesis of the metabolites takes place in plastids (terpenoids) or involves them (phenylpropanoids). Typical examples are medicinal plants and herbs of pharmaceutical importance such as mint (Mentha sp.) and jewel orchid (Anoectohilus sp.). However, a decrease in secondary metabolites, flavonoids and phenolics, was also observed with increasing irradiance in the medicinal plant cat’s whiskers (Orthosiphonstamineus), indicating that the light irradiance may have negative consequences on secondary metabolite production. In higher plants, it has been documented that depending on species and growing conditions, the secondary metabolites and pigments in the flavonoid family accumulate under photoinhibitory conditions at cell level, although the mechanistic aspects of LED light effects are not well understood.
The high fluence effect of LED light has been studied more in photosynthetic microorganisms, partly because they present huge biotechnological and economic potential (biofuels, pharmaceuticals, food additives and cosmetics). For instance, Wang et al. assessed the economic efficiency of energy converted to biomass in microalga (Spirulinaplatensis) culture under different LED monochromatic lights as grams of biomass per litre per dollar. The data showed that at the light intensity of 1500–3000 µmol m−2 s−1, red LEDs consumed the least power and yielded the highest economic efficiency when emitted at the same intensity compared with blue LEDs (up to 110 versus lower than 10 g per litre per dollar, respectively). However, such a high fluence is not always requested. For instance, in the green microalga Dunaliellasalina, light stress to drive the accumulation of β-carotene was within the range of 170–255 µmol m−2 s−1 using LEDs, whereas 1000 µmol m−2 s−1 photon flux was needed using conventional lights such as fluorescent lamps and high-pressure sodium lamps. Additional red or blue (470 nm) LED light caused stress whereby the xanthophyll cycle was activated. The additional blue light was less stressful than the red light. Katsuda et al.reported that red LED light allowed the growth of the green alga Haematococcuspluvialis, whereas blue LED light enhanced astaxanthin production. More recently, Katsuda et al.showed that in mixotrophic growing conditions, flashing LED light (8 µmol photon m−2 s−1) triggered similar astaxanthin concentration to continuous LED light (12 µmol photon m−2 s−1). Such low light requirement suggests the involvement of photoreceptors. A putative transduction mechanism of the blue light signal would involve major carotenoids in D. salina. Signalling of secondary carotenoid synthesis involves chloroplast-generated ROS. Much more investigation is needed to understand the impact of LED light on primary and secondary metabolism of photosynthetic organisms.
Modification of the metabolism through supplemental monochromatic lighting: The effect of supplemental blue and/or red LED light is not limited to growing and developmental properties. They also increase the antioxidant content of vegetables. For instance, red (658–660 nm) LED light increased the phenolics concentration in lettuce leaves and the anthocyanin content of red cabbage leaves. One can therefore imagine designing supplemental LED light treatments as pre- or post-harvesting processes to fashion raw materials. This would provide great commercial and production advantages. For instance, Colquhoun et al.used LED treatment to modify the synthesis of volatile compounds in flowers and fruits. In tomato, a red LED treatment (668 nm, 50 µmol photon m−2 s−1) triggered a significant increase of 2-methyl-butanol and 3-methyl-1-butanol levels, whereas the amount of cis-3-hexanol was reduced when compared with the levels reached with white LED light. Because two of those three compounds are involved in the degree of tomato sweetness, one can hypothesize that the LED treatment will impact the taste of the fruit. The mechanism of action of the monochromatic light has not been studied as yet, but one can assume that the red light affects terpenoid production in the chloroplast through phytochrome. Alternatively, specific ROS production could have the same action as shown in the case of secondary carotenoid synthesis
A CASE STUDY OF LED LIGHT FOR OPTIMIZATION OF PLANT
GROWTH AND INCREASING YIELD
Greenhouses have been introduced into commercial crop production for several decades. Currently, small industries and individuals have been involved in urban farming with success in producing fresh food, in a sustainable way, able to deliver to the final consumer in an instant, without transportation costs or storage needs. Also, people that encounter these kinds of businesses and taste the products tend to prefer them, because they are healthier, fresher and last much longer than the imported equivalents. Light is one of the most important factors in plant growth development. Light is not only the energy for driving photosynthesis, but an essential signal to mediate downstream gene expression of substance metabolism for the plant to acclimate to environmental fluctuation (Chen et al.2004)
In the commercial greenhouse, light supplementation using artificial light can significantly increase crop yield and nutrition quality, especially in low-light-intensity seasons like winter and late autumn (Lu et al., 2012; Yorio et al., 2001). With the development of urban agriculture, artificial light has become the most important way to control the light conditions. For a long time, people were using fluorescent lamps, filament lamps and high-pressure sodium lamps (HPL), and much research was carried out to test their effects (Tibbitts et al., 1983). However, these kinds of light tend to consume large amounts of electrical energy and release a lot of heat (which will also increase the cooling system cost), and their spectra are not very suitable for plants, which leads to excessive waste of energy (Randall and Lopez, 2014). The most important element in controlling artificial farming costs is supplying light for photosynthesis and growth by light sources with high photoelectric efficiency. Light-emitting diodes (LEDs) have been proposed as alternative light sources in controlled agricultural environments since, compared with traditional horticulture light sources (e.g., HPL), LEDs have drastic advantages, such as superior lifetime, reduced size, cooler emitting temperature, and reduced energy consumption (Massa et al., 2008). An exciting potential of using LED lighting is the development of species-specific light recipes comprising the optimum proportion of specific narrow-band wavelength light that can optimize plant growth, development and other desirable traits (e.g., increase phytochemical content) (Bian et al., 2015, 2016), whilst significantly reducing the energy input compared with traditionally used horticulture light sources. Recently, the effect of LEDs on plant growth and development has aroused increasing interest. However, the results of related studies are sometimes different, and even contradictory (Avercheva et al., 2009; Bian et al., 2016; Hogewoning et al., 2010; Urbonavičiūtė et al., 2007). A hypothesis has been raised that the different application strategies might arouse different responses of plants to LED light treatments. We conducted experiments using different light spectral compositions and application strategies of LEDs to further reveal the disadvantages of LEDs in energy saving and plant nutrition quality improvement.
MATERIALS AND METHODS
Plant material and growth conditions
1. Experiment I.
Pak choi (Brassica rapa ‘Bonsai’, Chinensis group) seeds were sown in commercial substrate and germinated in an environment-controlled growth chambers. After germination, seedlings were randomly treated with red (650-670 nm; R) and blue (455-475 nm; B) LEDs (Green power research module, Philips) with four different R/B ratios for 14 days. The spectral compositions of light treatments are summarized in Table 1. The photosynthetic photon flux density (PPFD) of all light treatments for pakchoi was maintained at 130 μmol m-2 s-1 through adjusting the distance between the light sources and plants. In the next step, pakchoi seedlings were grown under mixed red and blue LED light, but with high (220 μmol m-2 s-1), medium (150 μmol m-2 s-1) and low (100 μmol m-2 s-1) light intensities to investigate light-use efficiency. Pak choi grow under fluorescent lamps (FL; Unigro, 12/6N) with a PPFD at 220 μmol m-2 s-1 were used as a control. Each treatment light had triple replications (four or five plants per replication). In the growth chamber, photoperiod, day/night temperature, relative humidity and CO2 level were set at 16 h, 25±1/20±1°C, 65±5% and 400±20 ppm, respectively.
2. Experiment II.
Tomato (Solanumlycopersicum) seeds were sown in commercial substrate and grown under FL (Unigro, 12/6N) with a PPFD at 150 μmol m-2 s-1. Every other day, tap water was added from the bottom of the pots to keep the substrate wet. When plants had their second true leaves, they were randomly treated with monochromatic red (650-670 nm, R) LEDs, monochromatic blue (455-475nm; B) LEDs, or mixed red and blue LEDs (R/B=1). Plantsgrown under FL were used as a control. Every other day, light intensity was monitored with a spectrometer (Skye Instruments Spectra Sense, RS 232). Light intensity for all the light treatments was kept at 200 μmol m-2 s-1 by adjusting the distance between the light sources and the top of the plants. Other environment factors were the same as in experiment I.
3. Experiment III.
Lettuce (Lactuca sativa L.) was seeded in plastic seedling trays filled with peat:vermiculite (3:1, v/v) and grown in a controlled growth chamber. Fluorescent lamps (T5, PHILIPS) were used as light sources for seedling cultivation and light intensity was maintained at 100 μmol m-2 s-1. The other environmental factors were set as in experiment I. When seedlings had their second true leaves, they were transplanted into a hydroponic growth system and grown under white (400-700 nm; W), LED light and combined red (peak at 660 nm; R) and blue (455-475 nm; B) LED light with/without green (515-545 nm; G). The light intensity and photoperiod were 200 μmol m-2 s-1 and 12 h, respectively and other environmental factors were as described in experiment I. Before harvesting, lettuce plants were conducted continuous light treatment for 24 h. The light treatment details are summarized in Table 2. These LED light sources (VQ-G200; Vanq Technology, Shenzhen, China) were maintained at 20 cm above the canopies of the plants. The PPFD of the LED light sources was monitored daily by a quantum sensor (LI-190SA; LI-COR, Lincoln, NE, USA)
. Plant growth determination
Pak choi seedlings were sampled after treatment with different light spectra for 7 days. Plants were cut from the hypocotyl. The shoots were weighed and then dried in an oven at 72°C to calculate shoot dry weight. Leaf areas were monitored using Li-3001C (LI-COR, Lincoln, NE, USA). To investigate energy-use efficiency, all plants were harvested to determine plant fresh biomass after 3 weeks of growth under different light intensities. The light-use efficiency was calculated as average fresh weight of plant divided by light source power used (kW h-1). Each measurement was repeated three times with three or four plants per replication
RESULTS
Effects of light spectral composition on pakchoi growth
Shoot fresh and dry weights and leaf areas were significantly affected by the blue light percentage in the light source (Figure 1A-C). The fresh and dry weight of shoots and leaf area were the highest under the 23% blue light treatment, followed by 27 and 100% blue light treatments. The lowest values of these parameters were obtained under the monochromatic red light (0% blue) light treatment. Furthermore, there was a significant linear correlation between fresh weight and leaf area of pakchoi.
Light energy-use efficiency under different light intensities
There were significant differences in plant fresh weight and light-use efficiency under different light intensities. Under the same light intensity (220 μmol m-2 s-1), the fresh weight of plants under red and blue LEDs was comparable to that of FL (control), but a decrease in light intensity of combined red and blue LEDs resulted in a significant decrease in shoot fresh weight. There was a significant difference in light-use efficiency between high light intensity and medium light treatment. Compared with FL, light-use efficiency increased by 55, 114 and 115% for mixed red and blue LEDs with PPFD at 100, 150 and 220 μmol m-2 s-1, respectively.
Photosynthetic performance of tomato under different LED lights
Tomato plants grown under the white LED light displayed significantly higher rates of photosynthesis than those grown under other LED light. Plants grown under monochromatic red and mixed red and blue light exhibited similar photosynthetic rates, but plants grown under monochromatic blue light displayed significantly impaired rates ofphotosynthesis compared with other spectral compositions of light. Stomatal conductance was highest under monochromatic blue light, followed by white, mixed red and blue, and finally monochromatic red light . Among different LED light treatments, there was no significant difference in Fv/Fm. The highest and lowest ΦPSII were observed under white and mixed red and blue LED light, respectively. However, there was no significant difference between monochromatic red and blue light treatment in tomato leaf.